Panagiotis L. Kastritis
Research
Cryo-electron microscopy of membrane-bound protein complexes
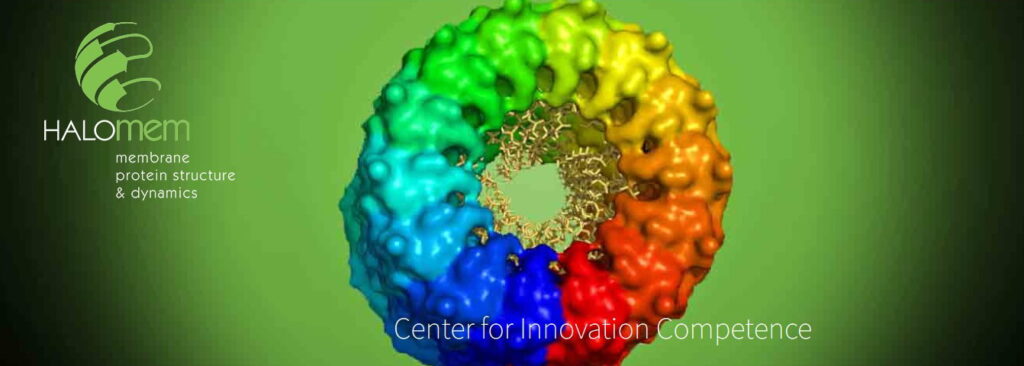
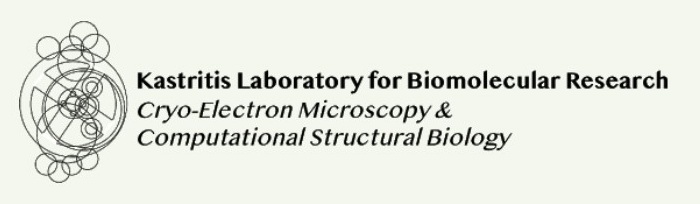
Research Vision of the Kastritis Laboratory for Biomolecular Research
Enzymopathies are metabolic disorders, often genetic, resulting from missing or defective enzymes. In order to prevent, combat or repair defective enzymes that lead to disease, it is vital that we understand the structure, function and interactions of enzymes and their complexes, ideally within their native cellular context.
In their native cellular environment, enzymes are neither isolated nor randomly distributed. Instead, they co-compartmentalize, and often transiently interact to form supercomplexes of metabolic pathways (commonly referred to as metabolons). The formation of metabolons allows the intermediate product from one enzyme to be passed directly into the active site of the next consecutive enzyme of the metabolic pathway. Their role and existence were biochemically known for years but due to their sheer size and transient nature, understanding their molecular organization remains a challenge. As a consequence, traditional structural biology methods have helped us in understanding the structure and function of their constituent enzymes but not their higher-order assembly and how their interfaces are formed.
My research vision is to understand the molecular architecture of metabolons by integrating structural, biophysical, biochemical and biocomputational methodologies. This multidisciplinary approach aims at elucidating the molecular mechanisms that underpin cellular respiration in atomic details. In the long term, these methodologies will allow discerning cellular metabolons by visualizing their components and examining the interactions between the molecules that form the assemblies.
This research will ultimately provide new crucial insights not only to cellular respiration but also an entirely new view of cell mechanisms in general. The unsolved mystery of temporal and spatial synchronization of a myriad of protein subunits to perform a specific cellular function may be rationalized by the formation of supercomplexes. These unprecedented entities provide a whole new avenue for targeted drug design. Rather than targeting the active sites of individual subunits -which share many physical and chemical characteristics thus compromising specificity- I envisage a therapeutic strategy that targets the interface between proteins in the supercomplex that are likely to be unique.
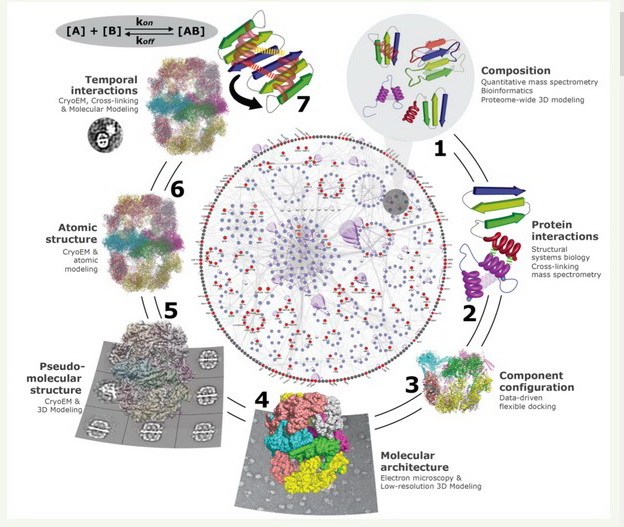
Abb. 1 Die Kombination von strukturellen, biochemischen und bioinformatischen Methoden ermöglicht das Verständnis einer zellulären Organisation von Proteinkomplexen bis hin zur atomaren Auflösung.
Instruments
High-resolution & screening Cryo-Electron Microscope Thermo Fisher Glacios cryo-TEM
We use the Glacios for screening and initial high-resolution cryo-electron microscopy studies of protein complexes and their higher-order assemblies.
High-resolution Cryo-Electron Microscope JEOL-JEM3200 FSC
We use the JEOL-JEM3200 FSC for high-resolution cryo-electron microscopy of protein complexes and their higher-order assemblies.
Cluster infrastructure
We use the IANVS high-performance computing cluster for high-end biocomputation of biomolecular complexes.
Selected Publications
*co-first author, *co-corresponding author
Top 5 Publications
Kastritis PL*, O’Reilly FJ* et al. Capturing protein communities by structural proteomics in a thermophilic eukaryote. Mol Sys Biol. 2017;13: 936.[The article was highlighted as the cover in Mol Sys Biol. We applied cryo-EM, proteomics, chemical cross-linking, network biology and computer modeling and were able to elucidate the structural basis of interactions within cellular extracts of C. thermophilum. Using the stability of the organism, we were able to resolve the high-resolution structure of fatty acid synthase at 4.7 Å from cell extracts and its involvement in a metabolon with a carboxylase. HALOmem can apply this method to understand enzymatic interactions in eukaryotic cells using biochemistry, computer modeling, electron microscopy and data analysis].
Visscher KM*, Kastritis PL*, Bonvin AM. Non-interacting surface solvation and dynamics in protein-protein interactions. Proteins. 2015;83(3):445-58.[This article describes molecular dynamics simulations on transient complexes to understand contributions of solvation and flexibility to the binding affinity. Distant effects from the outer surfaces contribute significantly to the biophysics of complexation for a variety of protein complexes. Simulations can be applied to modelled interfaces of cellular metabolons to understand biophysical contributors to weak interactions between enzymes and how these change upon mutation or between species].
Kastritis PL et al. Proteins feel more than they see: fine-tuning of binding affinity by properties of the non-interacting surface. J Mol Biol. 2014;426(14):2632-52.[In this seminal work of our group (cited in F1000 as exceptional), we analyzed biophysical contributions to the binding affinity of >150 transient complexes with experimentally-verified binding affinities. We determined several novel contributors, mainly long-range electrostatics and solvation effects stemming from the non-interacting surfaces of these interactions. Such calculations and the model proposed can be applied to understand the weak nature of enzyme complexes involved in higher-order assemblies in metabolism and quantitatively calculate their (native and mutant) binding affinities, predicting which interactions are more stable].
Kastritis PL, Rodrigues JP, Bonvin AM. HADDOCK(2P2I): a biophysical model for predicting the binding affinity of protein-protein interaction inhibitors. J Chem Inf Model. 2014;54(3):826-36.[In this article, we derived a robust model to predict the binding affinity of small molecules that aim to disrupt or modulate protein-protein interfaces. Therefore, this program and the developed scoring function can be applied for computational structure-based design of small molecules to e.g. affect metabolon formation in various metabolic pathways].
Kastritis PL et al. Solvated protein-protein docking using Kyte-Doolittle-based water preferences. Proteins. 2013;81(3):510-8.[A robust, flexible docking simulation has been developed in which the solvent is explicitly considered. Proteins are flexibly docked within their solvation shells and hydrophobic effect is simulated by randomly displacing water molecules from the formed interfaces using a hydrophobicity potential I derived from amino acid biophysics. This protocol can be directly used to dock and consequently understand binding modes of metabolons, where solvent effects in such transient interactions must play major roles].
Group Members
Group Leader
Dr. Panagiotis L. Kastritis
Jun.-Prof., Ph.D.
Senior Scientist
Annette Meister
PD Dr.
Staff Scientist
Fotios Kyrilis
MSc. Molecular Medicine
Staff Scientist
Dr. Farzad Hamdi
Ph.D. Materials Science
Post-Doc
Dr. Dmitry A. Semchonok
Ph.D. Biochemistry
Scientist
Christian Tüting, M.Sc.
M.Sc. Biochemistry
Ph.D. Candidate
Lisa Schmidt, M.Sc.
M.Sc. Molecular Biology
Ph.D. Candidate
Ioannis Skalidis, M.Sc.
M.Sc. Systems Biology
Ph.D. Candidate
Kevin Janson, M.Sc.
M.Sc. Biology
Links
The Kastritis Lab blog & website:
https://blogs.urz.uni-halle.de/kastritislab/
Full list of publications